Introduction
Intraoperative neuromonitoring is a clinical neurophysiology procedure, most often equated with verification of nerve impulse transmission in the structures of the spinal cord during spinal surgery, not affecting the aseptic nature of the surgical field. It is also often used to assess the neuronal function of the supraspinal centers in neurosurgery and surgical reconstructive procedures in the peripheral nervous system.
Currently, “neuromonitoring” is also more and more often used in terms of the use of comparative clinical neurophysiology examinations to reliably quantify the progress of treatment, including rheumatology, neurology, neurosurgery, orthopedics and rehabilitation.
The main aim of classical intraoperative neuromonitoring, since the development of its methodological principles of stimulation and recordings, was reducing the risk of possible neurological complications associated with spine surgery, most often in patients with idiopathic scoliosis [1, 2].
The success of spondyloorthopedic treatment in cases of patients with spinal deformities depends on many factors, including the lack of iatrogenic impact of the surgery itself in relation to nerve structures.
The procedure of neuromonitoring includes verification of the nerve impulse transmission in the motor and sensory pathways of the spinal cord as well as the spinal roots using neurophysiological procedures with electrical or magnetic stimuli.
According to the report of the Scoliosis Research Society from 1974, in about 100 out of 8,000 patients, the treatment was accompanied by complications related to changes in sensory and motor neural transmission of the spinal cord pathways.
In 2010 Carl et al. [3] presented a study of 700 spine cases treated surgically and identified a 14% incidence rate of perioperative adverse events, which resulted in a final 3.2% complication rate.
Data from PubMed in 2020 provide the incidence rate of perioperative adverse events in scoliosis patients at 8.2%. The development of surgical techniques reduced the incidence of complications. One of the most popular methods of verifying non-invasive treatment until the end of the 1980s was the Vauzelle and Stagnara wake-up test.
During this test, the integrity of the spinal cord motor pathways is verified. Apart from the negative psychological aspects in relation to the patients tested with this method, its effectiveness is not unequivocal [4].
The method of somatosensory evoked potentials (SEPs), from the very beginning of its clinical application for verification of the afferent pathways’ transmission, was burdened with the instability of the recording parameters (both amplitude and latency) at the successive stages of the surgical procedures and the influence of the level of anesthesia on their fluctuations. In some cases, the inability to record SEPs came from a reduction in the responsiveness of nerve structures to stimulation with an electrical stimulus resulting from the possible process of ischemia [5].
In 1980, Merton and Morton, during a neurosurgical operation, successfully introduced the technique of intraoperative recording of motor evoked potentials (MEPs) using transcranial stimulation, first with an electrical stimulus and then with a magnetic stimulus, which turned out to be effective enough to overcome the skepticism of surgeons regarding its usefulness [6].
Intraoperative neuromonitoring at the beginning of the 21st century is not only a standard in which surgeons see the potential benefit of increasing the safety of the patient’s treatment; it is also a strong point of arguments of hospital administrators in negotiations with lawyers representing the patient [7].
The introduction of neuromonitoring procedures to many countries in Europe was justified by the statistics of the decrease in iatrogenic perioperative complications. Since 2010, in accordance with the recommendations of the American Society of Neurophysiological Monitoring, clinical neurophysiologists have more and more often supported surgeons in performing this procedure [8].
In pediatric spine surgery, including the correction of juvenile or adolescent idiopathic scoliosis, during neurophysiological monitoring more and more attention is paid to enhancing the precision of the measurement of nerve impulse transmission in the spinal cord pathways, and, on the other hand, reducing the risk of invasiveness of the applied stimulation and recording methods. A novelty in this issue is the possibility of recording potentials using surface electrodes instead of the standard needles.
Recordings of MEPs with surface electrodes instead of needle electrodes including muscle and nerve combinations during neurophysiological monitoring associated with surgical interventions to the spine begin to make sense because of anesthesiological influences and pediatric purposes. The occurrence of complications after implantation of subcutaneous needle electrodes for transcranial stimulation or recording from muscles in the form of ecchymoses, bruises or rare infections was reported [9].
Especially in children, in 16% of patients, in whom MEP recordings using needle electrodes were made, reddening of the skin was observed, with the accompanying symptom of increased pain, sometimes lasting up to 6 months after the surgery [10].
Darcey et al. [9] demonstrated a significant percentage of the nerve damage during direct contact with a needle electrode inserted into the muscle for purpose of neurophysiological neuromonitoring.
Tamkus and Rice [11] demonstrated the occurrence of complications in the form of burns from puncture needle electrodes used for recording during neuromonitoring, when intraoperative magnetic resonance procedures were used in parallel. The same authors also indicated a significant risk of needle stick injuries in neurophysiologists and other workers in the operating room during electrode implantation and removal after surgery.
The quality of MEP recordings during intraoperative neuromonitoring from muscles can be significantly influenced by the depths of anesthesia or muscle relaxant administration, but not those recorded from nerves [12].
It refers to both the phenomena of the decrease of neuronal transmission along ascending and descending tracts at spinal and supraspinal levels as well as the transmission of acetylcholine released at the level of the neuromuscular junction. The influence of the latter can be omitted by recording MEPs directly from nerves additionally to the recording from muscles during surgical procedures.
There is a common agreement on the practical significance of MEPs recorded following transcranial electrical stimulation during surgical procedures, especially when the MEP recording station is located far beyond the exposed spine.
The aim of the study is to review the practical usefulness of MEPs in clinical diagnostics and present the possibilities for modifications increasing non-invasiveness, safety and diagnostic precision.
Material and methods
Participants
Our preliminary results on the possibilities to modify or improve the MEPs recorded pre- and intraoperatively are based on observations performed in 280 sessions in patients undergoing the surgical correction of idiopathic scoliosis (267 girls aged 8–18 years and 13 boys aged 13–17 years) (Table I).
Table I
Demographic and anthropometric characteristics as well as scoliosis type and curvature magnitude based on X-rays of the patients treated surgically
Variable | Age [years] | Height [cm] | Weight [kg] | BMI | Lenke’s scoliosis type* | Cobb’s angle* |
---|---|---|---|---|---|---|
Patients 267 F, 13 M N = 280 | 8–18 14.7 ±2.3 | 138–179 154.2 ±2.9 | 42–78 53.7 ±7.6 | 18.5–29.3 23.4 ±3.2 | A = 112 B = 108 C = 60 | Primary 42–85 56.1 ±6.8 Secondary 32–42 37.3 ±2.6 |
The experiences of using “real-time monitoring” in 35 cases of patients allowed for evaluation of the utility of this method with respect to the total duration of surgical procedures.
Neuromonitoring procedures
Preoperative neurophysiological studies included recordings of MEPs following transcranial magnetic stimulation while recordings were performed bilaterally from peroneal nerves and anterior tibial muscles (Fig. 1 A, B, D, E).
Fig. 1
Photographs presenting principles of MEP methodology recorded pre- (A, B, D) and intraoperatively (C, E–G). Preoperative evaluation of the patient’s neurological health status includes MEP bilateral recordings with the bipolar surface electrodes (D) from proximal and distal muscles as well as the peroneal nerve branches along their anatomical passage at the knee area following the transcranial magnetic stimulation of the motor cortex (A). “Hot spots” for the intraoperative bipolar electrical stimulation (C) are marked on the head (B) exactly in the same places for evoking preoperatively the maximal MEP amplitudes. Intraoperatively MEPs are recorded with pairs of surface or needle electrodes (secured with sterile tape) from nerves or muscles of lower extremities (E). The neuromonitoring device in the operating theatre (F, left side) is far from the operative area (G, exposed dorsal aspect of the spine from cervical to lumbosacral levels in one of the patients undergoing scoliosis correction).
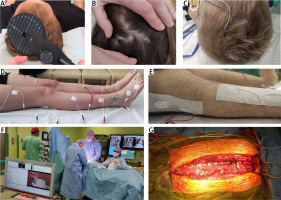
Motor evoked potentials were recorded using the 8-channel KeyPoint Diagnostic System (Medtronic A/S, Skovlunde, Denmark). Standard disposable Ag/AgCl surface electrodes with an active surface of 5 mm2 were used.
The active electrode was placed on the muscle belly and the reference electrode on its distal tendon according to the guidelines of the International Federation of Clinical Neurophysiology – European Chapter. When recordings were obtained from the muscle and while recorded from nerves, the active electrode was placed more proximally than the reference electrode (Fig. 1 D, E).
The ground electrode was located on the leg, near the knee. The recorder’s low-pass filter was set to 20 Hz, the high-pass filter to 10 kHz, the time base at 10 ms/D, and the amplification of signals was set between 200 and 5,000 µV. A bandwidth of 10 Hz to 1,000 Hz and digitalization at 2,000 samples per second and channel were used during recordings.
The resistance between the surface of the electrode and the skin was decreased with electroconductive gel. The examination was performed in an air-conditioned room with a controlled temperature of 22°C, in a supine position. The MEPs induced with a magnetic field were induced transcranially with a single, biphasic, 5 ms pulse to evaluate the efferent transmission of neural impulses from the spinal motor centers to nerves above their anatomical passage and the respective effectors innervated by them.
Motor evoked potentials were induced using a C-100 circular coil, with 110 mm diameter, connected to a MagPro X-100 pulse generator (Medtronic, Denmark). The maximum limit of the magnetic field stimuli was 2.4 T on the skin surface. The stream of the magnetic field elicited with the coil at the strength 70–80% of resting motor threshold (RMT; 0.84–0.96 T) excited all neural structures up to 3–5 cm deep.
The final averaged recording was obtained from at least 3 stimulations performed using a single magnetic pulse with a strength of 60–75% of the maximal stimulus output. The parameters of amplitudes and latencies of MEPs recorded from nerves and muscles were output measures. The amplitude was measured from peak to peak of the signal, the latency from the stimulus application marked by the artefact in the recording to the onset of the positive inflection of potential, the duration from the onset of the potential to its end with reference to the isoelectric line [13].
The patients did not report the stimulation as painless, but they felt the small spread of current to the lower extremities; they were always awake and cooperative.
Intraoperative recordings were performed with the ISIS system (Inomed Medizintechnik, Emmendinger, Germany) (Fig. 1 C). Motor evoked potentials were induced as a result of transcranial electrical stimulation or magnetic field stimulation in areas of the cortical motor fields for innervation of the thumb and selected muscles of the lower extremity (Fig. 1 B) by a sequence of 4 to 6 pulses (duration of a single pulse 500 µs) with a maximum intensity of 200 mA via bipolar screw electrodes or less frequently needles (Fig. 1 C) [14].
The recorded potentials were characterized by a variable amplitude from 500 to 5,000 µV and latencies in the range from 17 to 40 ms depending on the conduction distance. Potentials did not require averaging. The following standard settings were entered for the MEP measurements: filters hardware high-pass [Hz] 30; software high-pass [Hz] 0.5; software low-pass [Hz] 2,000; stimulation frequency [Hz] 0.5–2.4 ms intervals.
Transcranially induced MEPs evoked extensive depolarization of the motor and sensory centers of the cerebral cortex. Stimulation with a magnetic field at 1.5 T or the electrical stimuli at 200 mA, due to the strength and influence of both magnetic and electric fields, should not be performed in patients with pacemakers and other electronic implants, episodes of epilepsy during treatment, significant disturbances in the vascular system, or symptoms of increased intracranial pressure. Neuromonitoring procedures in infants and pregnant women are not recommended.
Before starting the surgery, after implanting the stimulating (Fig. 1 C) and recording (Fig. 1 D, E) electrodes in the supine position of the patient, the electrodes’ impedances should be checked, the correct values for needle electrodes should be in the range from 0.1 to 5.0 kΩ, indicating correct connections with the recorder amplifier.
The impedance of the stimulating electrodes distributed with the 10–20 system, inserted under the skin over the skull, should be around 0.8 kΩ, and the surface disposable bipolar recording electrodes from muscle groups or nerves along their anatomical passage should be in the range from 10 to 40 kΩ.
After the patient was transferred to the operating table in the prone position (in the case of posterior access to the spine) or in the position on the side (in the case of the anterior approach), the MEPs with reference amplitude and latency values were recorded as 0 s (reference values) for comparison with those which were recorded in the subsequent stages of the surgery (Fig. 1 G).
Amplitudes (in µV) and latencies (in ms) of MEPs were the outcome measurements undergoing the analysis. All results of MEPs obtained in 280 patients intraoperatively were also obligatorily compared to those preoperatively recorded following the magnetic stimulation, aiming to verify the neurophysiological status of the neural efferent impulses’ transmission.
Statistical analysis
Statistical data were calculated with Statistica 13.3 software (StatSoft, Kraków, Poland). Descriptive statistics included minimal and maximal values (range), mean and standard deviations (SD) for measurable values.
The Shapiro-Wilk test was conducted to assess the normality of distributions, as well as Leven’s test to measure the homogeneity of variances in some cases. The comparison of results from neurophysiological studies was performed with a dependent Student’s t-test (paired difference t-test). Values at p ≤ 0.05 were considered to be statistically significant.
Bioethical standards
This study was conducted according to the guidelines of the Declaration of Helsinki. Approval was also received from the Bioethical Committee of the University of Medical Sciences in Poznan, Poland (including studies on healthy people) no. 942/21. Informed consent was obtained from all the subjects involved in the study. All data generated or analyzed during this study are included in this published article.
Results
Our preliminary observations on 280 scoliosis patients lead to the conclusion that MEPs recorded from nerves do not undergo fluctuations at different stages of scoliosis corrections and the anesthesia effect more than MEPs recorded from muscles (Fig. 2 B and Table II).
Table II
Comparison of the results from MEP recordings performed pre- and intraoperatively in patients with scoliosis. Cumulative data from the left and right sides are presented (mean values and standard deviations are presented). Bold letters indicate significant differences at p ≤ 0.05
Fig. 2
Examples of intraoperative MEP recordings from two patients (A and B) undergoing surgical correction of idiopathic scoliosis. The arrangement of recording sites from muscles and nerves bilaterally presented in B is common for all examples. Calibration bars for amplifications (in μV) and time bases (in ms) are the same for each MEP recording. Following the transcranial electrical stimulation of supraspinal motor centers, similar parameters of latencies can be observed in MEPs recorded both with the needle (a, NE) and the surface (b, SE) electrodes except the lower amplitudes, but the shape of potentials remains unchanged (A). Comparison of the amplitudes in MEP recordings with surface electrodes (SE) especially from nerves before (a) and after (b) intensifying the level of relaxation convinces about the resistance to anesthetic conditions (B).
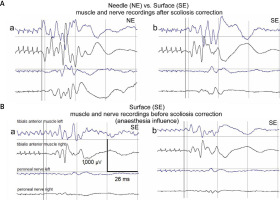
Mean amplitudes of MEPs recorded from peroneal nerves (274.2 µV) in comparison to those recorded from tibialis anterior muscles (1,280.1 µV) were about 4 times smaller. The mean latencies recorded from peroneal nerves (25.7 ms) were about 3 ms shorter than those recorded from tibialis anterior muscles (28.9 ms).
The results of the amplitude and latency parameters’ analysis in the potentials recorded before and after the correction of scoliosis indicated the usefulness of non-invasive surface recording, with the principle of its precision in determining the neural transmission in the spinal pathways (Fig. 2 A).
Motor evoked potential amplitudes recorded with surface electrodes (SE) had values less than half lower than those recorded with needle electrodes (NE). The average amplitude recorded from tibialis anterior muscles with the SE method was 364 µV, while the corresponding amplitude with the NE method was 792 µV (at p = 0.01); no differences in latencies of MEPs recorded with two methods were detected (at p = 0.06).
The immediate improvement of the efferent neural transmission in spinal pathways following scoliosis surgery has been expressed in the increase of the MEP amplitude parameter recorded for comparison before and after surgery at p = 0.04 (Table II).
No significant differences of the MEP latency parameter have been found. We observed that the use of non-invasive surface electrodes during neuromonitoring for MEP recordings shortens the average total time of pediatric surgical procedures from 5.5 hours to 4.5 hours at p = 0.04.
Discussion
The increase of the MEP recordings’ latency at 2–3 ms during the preparation of the surgical field with cauterization of muscles, observed in 35 out of 288 analyzed cases, may indicate the “warming effect” and reaction of the spinal cord structures, which is transient after cooling with 0.9% NaCl (at the temperature of 36ºC).
It should be remembered that during prolonged neurosurgical procedures, natural, gradual attenuation of the signals may occur, more in children than adults, and the origin of these changes remains unexplained [12]. The gradual decrease of MEP amplitudes at 10–30% bilaterally may occur during the correction of pathological lateral spinal curvature and the derotation of the spinal deformity.
The difference of 50% decrease between the reference value and the amplitudes recorded uni- or bilaterally (during pedicle screws’ implantation, corrective rods’ implantation, correction, distraction and derotation procedures of the spine curvature) is critical, indicating the blockade of the efferent spinal pathways neural transmission.
The proposed definition of “real-time” neuromonitoring comprises the immediate warning from a neurophysiologist about the changes in the patient’s neurological status during scoliosis surgery (especially during pedicle screws’ implantation, corrective rods’ implantation, correction, distraction and derotation of the spine curvature) exactly during the successive steps of corrective procedures (Fig. 3).
Fig. 3
Examples of “real-time neuromonitoring” bilateral MEP recordings from muscles and nerves of lower extremities during certain steps of the scoliosis surgery. Preparation of the surgical field with cauterization of muscles (A), pedicle screws’ implantation (B), corrective rods’ implantation, correction, distraction and derotation of the spine curvature (C). Calibration bars for amplifications (in μV) and time bases (in ms) are the same for each MEP recording.
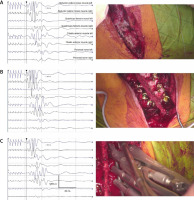
This is possible due to the simultaneous observation of MEP recordings and the camera image of the surgical field. We observed that this procedure shortened the average total time of the analyzed 35 surgical scoliosis corrections by about half an hour.
According to the assumptions of Malhotra et al. [8], neuromonitoring should be used in every surgery in which the surgeon foresees a real iatrogenic risk of the applied procedures for the nervous structures in the central and peripheral systems, and in the optimization of successive stages of surgical procedures.
The success of neuromonitoring during surgical operations is largely influenced by the understanding and meaningful cooperation between the team consisting of the surgeon, the neurophysiologist and the anesthesiologist [6, 15].
The use of surface electrodes during neuromonitoring for MEP recordings reduces the total time of surgery, both in terms of implantation and removal of needle electrodes instead.
In patients undergoing lateral curvature correction by about 30–40 degrees, it should be expected that the MEP recording method with surface electrodes is sufficiently precise when the amplitudes of recording in the preoperative examination conditions are in the range of 200–2,000 µV. It is also expected that the costs of SE neuromonitoring against NE may be reduced about eight times.
The method of intraoperative MEP recording from nerves versus muscles following transcranial electrical stimulation in patients undergoing scoliosis surgery is resistant to the anesthetic conditions such as the depth of anesthesia or relaxants’ administration.
Real-time neuromonitoring shortens the average time of surgery by about half an hour, and decreases the number of questions from the neurophysiologist to the surgeon and vice versa, resulting in an increase of the surgeon’s attention to the applied procedures to the spine.
Real-time neuromonitoring also increases the confidence of the surgeon in the patient’s health status during the surgery, and provides the possibility of external expert participation in the monitoring session.
Conclusions
Considering the issue of health care management, the above presented intraoperative neuromonitoring methods significantly affect the safety of the performed spondylo-orthopedic procedures.
Since the use of neuromonitoring not only before and after the treatment, but, what is very important, intraoperatively, it has been found that the safety of the pediatric patient has greatly increased.
From the point of view of the Hospital Management Board, the procedure clearly increases safety and limits financial claims resulting from possible complications.