Introduction
Systemic lupus erythematosus (SLE) is an autoimmune disease including both complex and multifactorial components. Systemic lupus erythematosus involves an interplay of environmental factors, genetic factors, sex hormones and factors relating to a deficient immune mechanism and is characterized by the production of antibodies which target the cell nucleus. The etiology remains unknown, but there are key clinical manifestations which are indicative of SLE, including inflammation, vasculitis, vasculopathy and deposition of immune complexes [1].
Sex hormones are also known as gonadocorticoids and include estrogen, progesterone and androgens. Their mechanism of action occurs via a nuclear receptor mediated signaling cascade. Sex hormones play a major role in sexual differentiation, but also exert an important effect on the immune system.
The influence that is implemented on the immune system results in sexual dimorphism. Females tend to produce “more vigorous cellular and more vigorous humoral immune reactions” with a higher resistance to particular infections and “suffer a higher incidence of autoimmune diseases” [2].
Both estrogen and testosterone may have an influence on the production of antibodies/autoantibodies. Testosterone has been shown to decrease the production of antibodies, therefore having an immunosuppressive effect, whereas estrogen increases the production of antibodies, which is immunoenhancing [2].
This enhanced capability of immune reactivity in females applies an effective resistance to infections, but on the contrary also enhances susceptibility to auto-immunity and immune pathogenic effects [3]. Estrogen impairs the process of negative selection of high affinity autoreactive B cells and modulates the Th2 response [3].
Additionally to its effect on B cells, estrogen also plays a role in the induction of T cell homing by increasing the homing marker CCR5 [3]. On the other hand, testosterone modulates the Th1 response and stimulates CD8 cells, while down-regulating several inflammatory factors and up-regulating anti-inflammatory factors.
On the other hand, residing in the human body we have bacteria, viruses and other microbes which comprise the human microbiome. The effect these microbes have on human physiology in both diseased and non-diseased states is enormous, and in healthy adults over 1,000 species of bacteria may be identified [4].
Research concerning the human gut microbiome has exploded in recent years with new information regarding its role in metabolism, immune defense and behavior surfacing [5]. There are several factors which influence the microbiome of the gut, including aging, environmental factors, diet, stress and exposure to certain medications [5].
For these reasons, the interplay between all 3 aspects is being clarified day after day and it is thus our interest to investigate the dynamic relationship of gut microbiota with sex hormones in SLE.
Sex hormones and gut microbiota
There is a bidirectional interaction between sex hormones and gut microbiota that contributes to sex-based variations in disease pathology, particularly in SLE (Fig. 1).
Fig. 1
Summary of the proposed interaction between sex hormones and gut microbiota in the pathogenesis of systemic lupus erythematosus.
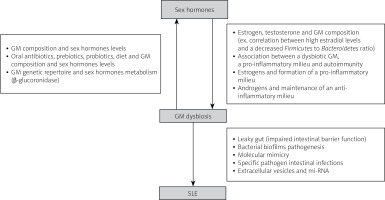
The feedback loop between gut microbiota and sex hormones affects autoimmunity by triggering either a tolerogenic or inflammatory effect. Investigating specific bacteria with pro-inflammatory or protective roles is needed to better understand these interactions [6].
Several studies have shown that the diversity of gut microbiota is influenced by various factors, including sex differences [7]. Certain bacterial strains, such as Erwinia, Allobaculum, and Anaeroplasma, were found to be more frequent in male mice than in female mice [8].
Testosterone levels in female mice were shown to increase when fecal microbiota was transferred from male to female mice. Hormonal replacement and gonadectomy can substantially alter the gut microbiota composition [9–12].
Major differences in gut microbiota composition between males and females are first seen following puberty onset and can be reversed after male castration. A correlation was observed between hyperandrogenemia and gut microbiota in mice with polycystic ovary syndrome [13–15].
Progesterone was shown to increase the growth of Prevotella intermedius and oral Bacteroides species in a cell-level study performed in the 1980s [16].
Estrogens
The level of estrogens is associated with specific gut microbiota (GM) composition, as shown in several studies on healthy men and women [17, 18]. For instance, an increase in estrogen metabolites correlates with a decrease in the Firmicutes to Bacteroidetes ratio and an increase in the Ruminococcaceae family in healthy women [17].
A direct correlation was found between high estradiol levels in healthy women and a decrease in the number of Firmicutes, and increase in the number of Bacteroidetes, and hence an overall decrease in the Firmicutes to Bacteroidetes ratio [19]
Moreover, there is a link between GM diversity and estrogen levels [20], with a strong association between alpha diversity and total estrogens in healthy men, but not in premenopausal women [18].
In women with polycystic ovary syndrome (PCOS), a correlation was observed between hyperandrogenemia and GM. These findings suggest that sex hormones play a role in shaping the GM and may contribute to sex-based variations in disease pathology.
Several studies performed in healthy women and men led to the finding that there is an association between the level of estrogen and specific GM composition [12, 17–21].
A positive correlation was found between the Subdoligranulum, Sutterella, Agathobacter and Collinsella genera and (E2) estradiol levels in obese women with PCOS [21]. However, a negative correlation was seen with Ruminococcus gnavus and Escherichia-Shigella genera [21].
Testosterone
A study conducted by Insenser et al. [20] showed a correlation between the abundance of the Raoultella genus and the ratio of total testosterone, free testosterone and free testosterone to free estrogen. Also, during the analysis of sex and sex hormones and their effect on GM genera, it turned out that Paraprevotella had an inverse association with estrogen and the strongest positive association with testosterone [20].
Sloan et al. [22] found a positive association between certain bacterial genera such as Escherichia/Shigella, Streptococcus, Alistipes, Rothia, Peptostreptococcus, Bacteroides, Blautia, Para bacteroides and testosterone levels in women with and without PCOS.
Also, an inverse correlation was found between certain genera such as Alistipes, Coprococcus, Akkermansia, Ruminococcus and Collinsella and testosterone levels [23].
Furthermore, a positive association was found between testosterone and the Prevotella genus in nonobese women with PCOS and obese women with PCOS [24]. However, a negative association was found between Eubacterium hallii group, Ruminiclostridium, Subdoligranulum, Lachnospiraceae, Ruminococcaceae, Tyzzerella and testosterone [24]. Moreover, a study was conducted on healthy men and a statistically significant association was found between the diversity of GM and testosterone levels [19].
Another study showed a significant association between the free testosterone to free estradiol ratio and alpha diversity (Shannon and Chaol indexes), and this correlation was also seen with the total testosterone level [20].
In addition, a negative association was found between diversity (PD and richness indexes) and testosterone levels when combining women with PCOS and healthy women [25].
Sex hormones, gut microbiota dynamics and molecular interactions
The genetic repertoire of the gut microbiota that are capable of metabolizing estrogens is called the estrobolome [26]. Estrogens are metabolized from their conjugate forms to their deconjugated forms via microbial secreted β-glucuronidase [27], then, by binding to estrogen α and β receptors, estrogens and phytoestrogens exert their effect and activate downstream intracellular signaling pathways, hence affecting gene expression [28].
The administration of oral antibiotics led to the increase of conjugated estrogen excretion lowering urinary estrogens [29–31]. Also, many in vitro and in vivo animal model studies show the importance of the estrobolome in the metabolism of estrogens [32–34].
Several studies have shown that probiotics and diet were able to alter β-glucuronidase activity, hence showing the importance of taking the diet and probiotics into account in further studies discussing the relationship between sex hormones and GM [35, 36].
Limited information is available regarding the molecular pathways and dynamics between GM and testosterone. The deconjugation of glucuronidated testosterone and dihydrotestosterone into their active/deconjugated form that will enter the bloodstream is facilitated by bacterial β-glucuronidase [37, 38].
Furthermore, in vitro conversion of glucocorticosteroids in the bile into androgens by Clostridium scindens may affect systemic and local testosterone levels in vivo [39, 40].
A dysbiotic GM can lead to a pro-inflammatory mucosal milieu which may lead to autoimmunity. As we previously mentioned, estrogen favors the formation of a pro-inflammatory milieu but androgens help maintain an anti-inflammatory milieu. Thus, diet and probiotics can alter the GM composition and diversity and can change the disease outcome, specifically in males [6].
Gut microbiota and systemic lupus erythematosus
Recently, a link between the gut microbiota and SLE has been proposed [41]. A breakthrough was reported Hevia et al. [42], showing that patients with SLE have restricted GM in comparison with twenty healthy counterparts.
Patients with SLE have an altered gut microbiota composition and microenvironment (differing from the GM of healthy individuals), including high presence of Actinobacteria, Bacteroidetes, and Proteobacteria and lower levels of Firmicutes in comparison with healthy controls [43].
It is important to note that SLE disease activity index is inversely associated with Firmicutes; hence lupus progression can be delayed by Firmicutes presence [44]. In addition, SLE patients have a decrease in the diversity and richness of GM in comparison with healthy controls [45, 46].
In studies done on mice, it was found that levels of Lachnospiraceae increased and Lactobacilli decreased in the GM of Murphy Roths large mice homozygous for the lymphoproliferation spontaneous mutation FASlpr (MRL/lpr) (more severe in females). Also, the intestinal colonization of Lactobacillaceae was negatively associated with SLE activity in mice and lupus progression is strongly correlated with Lachnospiraceae in MRL/lpr mice [47, 48].
Pathways linking GM dysbiosis and SLE pathogenesis include leaky gut (impaired intestinal barrier function), imbalance of the immune system, molecular mimicry, biofilms, sex hormones and others.
Leaky gut hypothesis
The intestinal mucosa has an intestinal barrier that protects it from bacteria, viruses, fungi, toxins, foreign and food antigens [49]. Calcium-containing protein from immune cells such as macrophages and neutrophils is a biomarker for damaged intestinal mucosa (impaired function). Stool samples from SLE patients contained high levels of calprotectin, hence revealing impaired intestinal barrier function [50, 51].
Moreover, many sera soluble markers such as lipopolysaccharides, CD14, and alpha1-acid glycoprotein were high in SLE patients, hence revealing the presence of intestinal bacterial translocation [46]. A study was conducted by Thim-Uam et al. [52] whereby a leaky gut was induced in lupus mice following administration of dextran sulfate solution.
The result was progression and aggravation of SLE disease in the mice because endotoxins and other chemicals were translocated out through the leaky gut, thereby promoting apoptosis [52].
In addition, anti-dsDNA autoantibodies and immune complex deposition were promoted by the leaky gut, leading to aggravation of the disease [53]. Also, after the administration of probiotics, antibiotics or diet change, the gut barrier and lupus were highly ameliorated in lupus mice [54, 55]. Hence, these previous studies confirm that damage to the intestinal barrier can lead or aggravated SLE.
Bacterial biofilm pathogenesis
A biofilm is a membrane that wraps bacteria and protects them from immune recognition and allows bacteria to develop drug resistance [56–58]. Salmonella typhimurium amyloid Curly fibers can bind to DNA in bacteria, hence forming biofilms and contributing to SLE pathogenesis [59].
After intraperitoneal injection of curli-DNA complexes in normal mice, antinuclear antibody (ANA) and anti-dsDNA autoantibodies were formed. Also, curli-DNA complexes cause proliferation of B and T cells and inflammatory monocytes and autoantibody production in lupus mice [59].
Molecular mimicry
When a particular structure of a microorganism is similar to a self-structure of the host, an autoimmune response can occur, leading to damage of host tissue. This is known as molecular mimicry [60]. Hence specific bacteria able to undergo molecular mimicry can lead SLE patients to form cross-reactive autoantibodies.
In a study conducted by Zhang and Reichlin [61], a bacterial antigen of Burkholderia and another transcriptional regulatory peptide were able to bind to dsDNA antibodies in SLE patients’ sera; this leads to the conclusion that the synthesis of anti-dsDNA antibodies in SLE patients is correlated with Burkholderia molecular mimicry.
A study showed that the molecular mimicry of environmental microbes or commensals enhances autoanti-body production in SLE, driven by HLA-DR restriction and T cells [62]. Furthermore, another study showed that mycobacterial cell wall glycolipids can bind to the dsDNA autoantibodies that are derived from SLE patients and mice [63].
An important factor in SLE pathogenesis is bacterial molecular mimicry. Stimulation of autoantibody synthesis can be through molecular mimicry. Also B and T cells are involved in the bacterial molecular mimicry process; however, limited information exists at present [64].
Specific intestinal pathogens
Many reports have associated the onset and progression of SLE and intestinal infections with specific pathogens. More studies are investigating the dynamics and pathogenesis of these specific pathogens.
One of these specific pathogens is Ruminococcus gnavus, which may be able to affect SLE disease progression. A study found an increase in sIgA-coated R. gnavus in stool samples from SLE patients, and the proliferation of the bacteria was directly proportional to the disease activity.
Also, an increase in the calprotectin levels in stool samples and in the lipopolysaccharides in sera was found in SLE patients, due to disrupted function of the intestinal barrier. Furthermore, this leaky gut leads to molecular mimicry and hence the synthesis of anti-dsDNA autoantibodies, hence aggravating SLE [46].
Another specific intestinal bacterial pathogen is Enterococcus gallinarum. The latter organism was found to have an important role in SLE pathogenesis. This bacterium damages the intestinal barrier and promotes Tfh (T follicular helper) and Th17 cell proliferation in F1 lupus mice. Subsequently, it translocates to the liver via the route of mesenteric lymph nodes and mesenteric veins. Also, it is able to enhance autoimmunity by triggering overexpression of ERV gp70 in the liver [51].
In addition, E. gallinarum was detected in liver biopsies of patients with autoimmune hepatitis and SLE, although it was not detected in non-autoimmune hepatitis patients or healthy controls. After the administration of a vaccine against this bacterium, the level of autoanti-bodies was reduced and bacterial translocation was inhibited [51]. This leads to the conclusion that therapy directed against these specific pathogens can suppress the autoimmune reaction.
Extracellular vesicle and miRNA
In a study, mice that were deficient in in IECs miRNA had GM dysbiosis and worsening of colitis, that improved after transplantation with fecal EV-derived miRNA from wild type mice [65]. This led to the conclusion that EV-derived miRNAs are able to control the GM composition and improve the progression of intestinal inflammation.
In addition, a recent study showed that the exosome miR181a relieves colitis by promoting GM homeostasis, decreasing the secretion of the pro-inflammatory factors and normal intestinal barrier function [66].
In summary, the EV-derived miRNA in the intestines can decrease or inhibit the progression of lupus by ameliorating GM homeostasis and intestinal barrier function.
Future perspectives
As we previously discussed, GM dysbiosis is correlated with the development of SLE and its progression. Many pathways and mechanisms can disrupt the homeostasis of the GM microenvironment and lead to GM dysbiosis and aggravation of the disease, such as leaky gut, molecular mimicry, specific pathogen interactions, biofilms, sex hormones, EV-derived miRNA and others.
Since SLE is a multifactorial heterogeneous disease, the therapeutic approach towards SLE via targeting the composition and the microenvironment of the gut microbiota could be a breakthrough. Administration of oral antibiotics could possibly be a future approach; however, symbiosis in other types of bacteria and increased bacterial resistance should be taken into account and studied in more depth.
Another approach could be via prebiotics or probiotics, hence aiming to construct and remodel the GM composition diversity in a way that would help create a bacterial homeostasis and an anti-inflammatory milieu by limiting activation of the adaptive immune system and the release of inflammatory markers and cytokines.
Vaccines directed against specific intestinal pathogens could be an approach, hence aiming to reduce the intestinal barrier damage, increasing its stability and preventing the translocation of organic molecules, antigens and other toxins into the bloodstream, and hence reducing the immune activation and thus suppression of SLE.
Furthermore, this can help prevent molecular mimicry induced by antigens similar to those of the host, hence decreasing immune activation, and can reduce complexes of biofilm proteins and DNA from triggering autoantibody synthesis.
The more we understand the complex pathways between the gut microbiota, sex hormones and the immune system, the more promising will be the novel therapeutic approaches.
Conclusions
This review of SLE pathogenesis reveals that this autoimmune disease is indeed multifactorial with inter-dynamicity of gut microbiota and sex hormones playing a major role in its onset and progression. Whether estrogen or testosterone have positive or negative correlations with the diversity and composition of the gut bacterial strains, this affects the intestinal barrier’s integrity, and hence alters the local immune microenvironment.
Moreover, the remaining mechanisms and pathways that link GM dysbiosis and SLE pathogenesis are numerous, and include leaky guy, bacterial biofilm pathogenesis, molecular mimicry, specific pathogen intestinal infections and extracellular vesicles and miRNA.